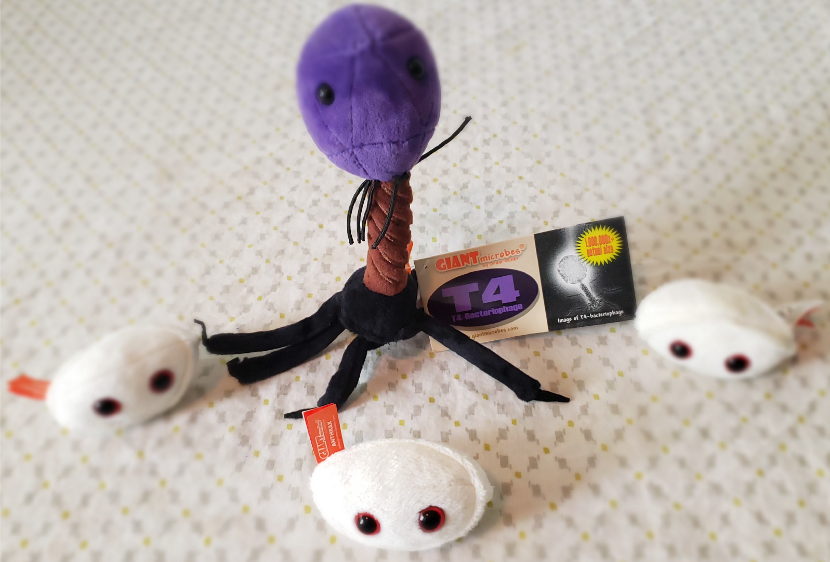
Phage and bacterial plush toys
British citizen Isabelle Carnell-Holdaway was born with cystic fibrosis, a genetic disease that causes buildup of thick mucus which interferes with the normal functioning of the affected organs, especially the lungs and digestive system. During her lifetime, she was chronically infected with an opportunistic bacterial pathogen, Mycobacterium abscessus, a relative of the bacteria that causes tuberculosis. Antibiotic treatments helped to keep the infection in check for almost eight years. In 2017, when she was 15 years old, she needed a double lung transplant. Prior to the surgery, she was treated with drugs to suppress her immune system so that her body wouldn’t reject the transplant. Following the transplant, however, the Mycobacterium infection resurfaced, and subsequent antibiotic treatments only worsened her condition. The doctors had lost all hopes of her survival, until researchers thousands of miles away at the University of Pittsburgh in the United States concocted a therapy to save the teenager’s life, the results of which were published in the journal Nature Medicine in May 2019.
So, what was the main ingredient of the magical therapy? Viruses. Yes, viruses! This may come as a surprise to many of us as the coronavirus pandemic rages through the world right now. We all blame the deadly coronavirus for everything bad that is happening around us today, and rightly so. So how could viruses cure Isabelle’s bacterial infection?
Well, the viruses that were used to treat Isabelle are special kinds of viruses called bacteriophages. Bacteriophages (or phages) are viruses that specifically infect bacteria and the scientists used them to their advantage to kill the harmful mycobacteria that were threatening Isabelle’s life. In this article, I will talk more about bacteriophages, how they infect their bacterial hosts, and recent progress made in the field of bacteriophage therapy and risks and uncertainties associated with it.
What are bacteriophages?
The word bacteriophage literally translates to “bacteria-devouring”. A bacteriophage can neither carry out any metabolic activity nor replicate on its own. So, it infects a bacterium and uses its cellular machinery to produce more of its progeny. Pretty much like a bacterial parasite. Phages are one of the most common and diverse biological entities on the planet. They are present in almost every known habitat, be it soil, ocean or your gastrointestinal tract and the current estimate of their population stands at ~10^31. This is greater than the total number of organisms on the planet, including bacteria themselves!
Phages come in different shapes, sizes and genomic content. We have only begun to scratch the surface of their diverse nature. The genetic material of phages can be either DNA or RNA, single stranded or double stranded, and can contain as few as four genes or as many as several hundreds of genes. The genome of the phage is enclosed in a protein shell called the capsid which ranges in size from 50-200 nm. You can think of the capsid as a plush ball and the stuffing inside as the phage genome. The ball can adopt different shapes, most common of which are icosahedral or filamentous. Many phages also have a tail which they use to attach to their bacterial host.
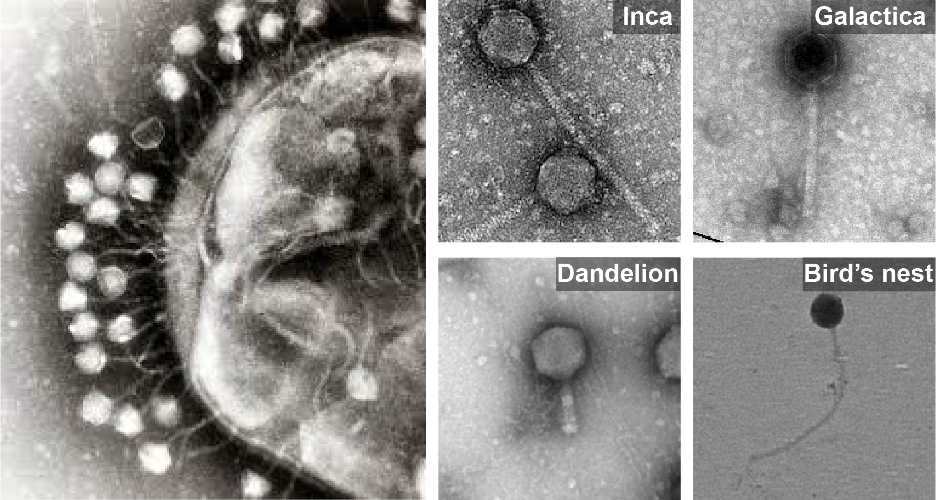
Left, image of phages infecting a bacterium (Source). Right, montage of electron microscopy images of phages taken by undergraduate students enrolled in SEA-PHAGES program. The fun part is that the students get to name the phage they isolate from the environment! The images shown here correspond to the phages Inca, Galactica, Dandelion and Bird’s nest.
How do phages infect bacteria?
By themselves, phages contain only limited genetic information. Hence, they largely depend on their host to supply precursor molecules and energy to replicate their genome and produce more of their kind. During infection, the phage first attaches to the bacterial host and injects its DNA into the cell. Following this, the phage can adopt one of two possible lifestyles as detailed below.
Some phages use up all the resources of their bacterial host for their replication, including bacterial ribosomes which now make proteins for phages rather than bacteria. Once a phage has made enough new phages inside the host, it will make proteins to poke holes in the bacterial membrane, causing the bacteria to lyse open in the process. The new phages generated can then explore their surrounding and search for new bacterial hosts to produce even more of their progeny in an exponential manner. Phages that replicate in this fashion are called lytic phages. On the other hand, some phages after infecting their bacterial host, do not immediately produce more of their progeny. Rather, they integrate their genome into the genome of their bacterial host. The integrated phage genome, referred to as the ‘prophage’ can copy itself every time the host cell itself replicates and divides. Phages that replicate in this fashion are called lysogenic phages. Phages can be lytic or lysogenic or sometimes switch between the two replication pathways depending on several external and internal influences. In either case, their aim is to make more of their kind, by hook (when they hijack the host cellular machinery as in lytic phages) or by crook (when they hitch a free ride on their host as in lysogenic phages).
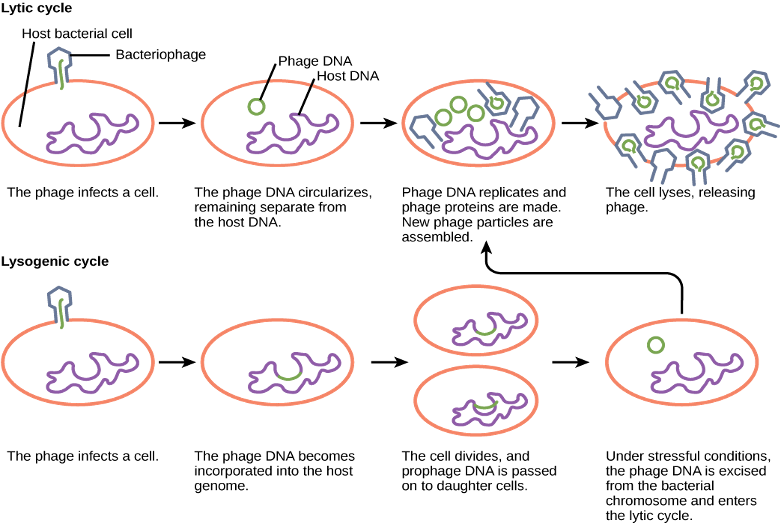
A schematic explaining the lytic and lysogenic cycle of phages inside their bacterial host (Source).
Let’s talk more about bacteriophage therapy
So now we have learnt that lytic phages have the potential to kill their bacterial hosts upon infection. What is amazing is that phages can be highly specific for their bacterial hosts, down to the strain level. Let’s take a simple example to understand this. While scientists were hunting for phages that could kill M. abscessus found in Isabelle’s body, simultaneously, they also tested these phages against M. abscessus isolated from a different patient. Even though the genomes of the two M. abscessus were highly similar, there were some differences in their sequences and the phages isolated against M. abscessus from Isabelle’s body did not work against M. abscessus isolated from the second patient. Unfortunately, the latter died as researchers were unable to find effective phages that could specifically kill the bacteria infecting the second patient in time.
The phenomenon that phages can be used to kill specific bacteria for therapeutic purposes, also called ‘phage therapy’, is not new. In fact, phages have been in widespread use to treat bacterial infections in Eastern Europe from when they were first discovered in the early twentieth century to even today. In 1919, Félix d'Herelle, one of the discoverers of phages used them to treat dysentery. However, the advent of the antibiotic era to fight bacterial infections pushed phages to the sideline. Now, with the increasing number of multi-drug resistant bacteria and pharmaceutical companies unwilling to spend billions of dollars to discover new antibiotics, phages have again come to the frontline as an alternative to antibiotics to fight pathogenic bacteria.
Like any other therapeutics, phages have their pros and cons. One of the advantages offered by phages over antibiotics is that they display higher target specificity. But this would also mean that specialized phages are needed for each patient which can be a fairly laborious task. Also, just as bacteria develop resistance to antibiotics, they can also develop resistance to phages. But we can avoid this problem either by isolating a new phage for the target bacterium (which is a lot easier and cheaper compared to finding a new antibiotic!) or use a cocktail of phages which would not only delay the onset of bacterial resistance but also increase the range of bacteria that can be targeted.
In the case of Isabelle, scientists used a three-phage cocktail against M. abscessus. The story behind how they obtained the three phages which were effective against the target is also very interesting. Prof. Graham Hatfull is the lead scientist at the University of Pittsburgh who spearheaded the phage therapy treatment for Isabelle. His lab and the Howard Hughes Medical Institute in the US launched a program called SEA-PHAGES in 2008 wherein every year undergraduates from over 100 institutes nationwide learn wet-lab and bioinformatic skills to isolate and characterize their own bacteriophages against a specific bacterial host from local soil samples. Hatfull and his team took advantage of >10,000 phages isolated against another Mycobacterium, M. smegmatis by students in the last decade and tested if they could identify a combination that would work well for Isabelle who was infected with M. abscessus. They identified one phage that kills M. abscessus highly efficiently and two other phages that infect M. abscessus with reduced efficiency. They then genetically modified the latter two phages to make them more effective. The doctors then administered the resulting three-phage cocktail to Isabelle topically, and within weeks her wounds and lesions started to heal. This was the first therapeutic use of phages to treat mycobacterial infection and the first one using genetically engineered phages! Previously, phages were also implicated in saving a 68-year-old diabetic patient infected with a multidrug resistant bacterial strain of Acinetobacter baumanii, where antibiotics failed completely. Recently, the United States Food and Drug Administration (FDA) has approved the application of phages in meat and poultry to kill bacteria causing food poisoning like Listeria monocytogenes.
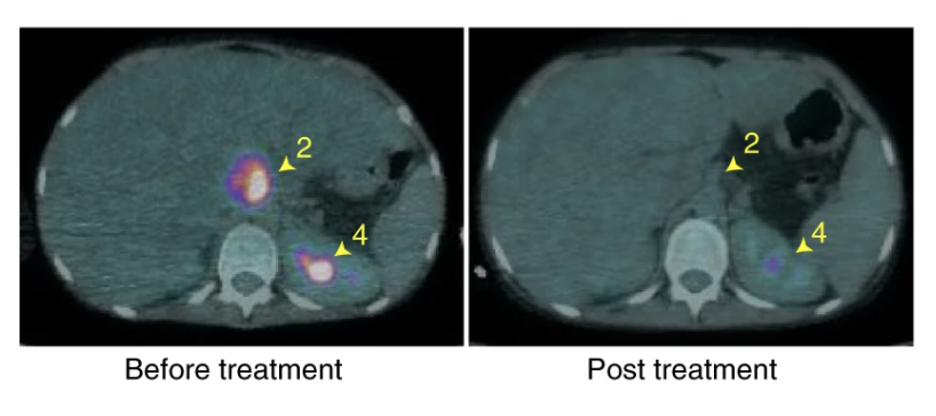
Isabelle’s CT scan 12 weeks before and 6 weeks after phage treatment. Arrow (2) points towards abdominal lymph nodes and arrow (4) towards kidney excretion. Significant healing was observed post phage therapy (Source).
Looking into the future, phages definitely hold a promise to treat notorious and recalcitrant bacterial infections, where antibiotics or other treatments may fail. However, the major roadblock which is currently preventing the widespread use of phages to treat bacterial infections is the lack of in vivo and in vitro data to understand their mechanism of action. Specifically, how do phages interact with our immune system and will they be safe for the patient in the long term? There is an ongoing debate in the field as to the exact mechanism of antibacterial activity of phages in the patient. Do phages kill bacterial cells directly or do phages first trigger an immune response in the host which then brings in molecules of our immune system to clear the bacterial infection? Finding answer to this question in patient and animal models is of critical importance. Specifically, if the latter mechanism holds true, phage therapy may not be effective in patients who have compromised immunity. Another consideration to keep in mind is that upon phage administration, our immune system can make proteins called antibodies which can then bind to proteins in phages in a lock-and-key manner to inactivate them. This may severely hamper the therapeutic activity of these phages by either clearing phages from the system or rendering them ineffective against their bacterial target. Moreover, we still don’t understand completely how these responses vary with the dose and time of phage administration.
At the moment, we lack large, controlled, randomized, double-blind clinical trials and studies in animal models to completely comprehend the safety and efficacy of phages. Hence, phage therapy is not approved as a standard treatment by the FDA. Currently, the FDA is approving phage therapy only on a case-by-case basis and that too, as a last line of defense for patients which have exhausted all other treatment alternatives. In Jan 2019, the FDA approved the first clinical trial of an intravenously administered phage therapy by University of California, San Diego School of Medicine. The trial will test phages for treatment of patients with ventricular assist devices (mechanical pumps that help pump blood in patients with fragile heart) infected by multi-drug resistant Staphylococcus aureus. Hopefully, future studies to understand the immunomodulatory effects of phages in animal models and comprehensive clinical trials will pave the way for phage therapies as a viable treatment option to save more Isabelles in the world.
Acknowledgements
I would like to thank Shruti Singla and Vu Le-Huy Tran for their thoughtful feedback and suggestions in improving the article.
Comments